Precipitation of Energetic Particles from Magnetosphere and Their Effects on the Atmosphere
Scientific rationale
There are various types of plasma waves in the Earth’s magnetosphere, such as whistler-mode chorus waves, hiss waves, magnetosonic waves, electromagnetic ion cyclotron (EMIC) waves, and electrostatic cyclotron harmonic (ECH) waves. Effects of these waves on energetic particles include acceleration and loss of energetic particles. They can scatter energetic particles in the ring current and radiation belts of the Earth and cause them to precipitate to the atmosphere [e.g., Shprits et al., 2008; Thorne, 2010; Li and Hudson, 2019]. The collision between these energetic particles and neutral particles in the atmosphere forms the aurora. An example of precipitated energetic electrons caused by chorus waves is shown in Figure 1 adapted from Kasahara et al. [2018]. Particles precipitated from the magnetospheres are sources of auroral particles and nitric oxide in the auroral region of the upper mesosphere and lower thermosphere (60-140 km), affecting atmospheric composition and dynamics over large areas [e.g., Sinnhuber et al., 2012; 2019].
Whether the loss of sub-MeV electrons can be caused by EMIC waves or not is a topic under debate in recent years. From theoretical aspect, in the quasi-linear approach under cold plasma approximation, setting upper cut-off frequency of EMIC waves close to the ion gyro-frequency can result in a resonance energy as low as ~100 keV. However, after taking hot plasma in the magnetosphere into account, the minimum resonance energy between EMIC waves and electrons is usually higher than 1 MeV [Cao et al., 2017]. Meanwhile, non-linear theory and related test particle simulations suggest that EMIC waves can cause fast precipitation of sub-MeV electrons. Some studies (e.g., Miyoshi et al., 2008; Capannolo et al., 2018) using observations from low-Earth orbiting (LEO) satellites report simultaneous precipitation of energetic electrons and protons and suggest that the precipitation of both populations is caused by EMIC waves. The radiation belt dropout event occurred on September 24, 2013 has been extensively studied. For example, using conjugate observations from Van Allen Probe A and MetOp-01 satellites, Capannolo et al. (2018) reported coincident observations of EMIC waves and precipitation of electrons with energies extending to tens of keV on September 24, 2013. By setting the upper cutoff frequency at 0.99 fcH and 0.99 fcHe for H-band and He-band EMIC waves respectively, Capannolo et al. (2018) calculated the quasi-linear pitch angle diffusion coefficients due to EMIC waves based on cold plasma approximation. Their results show that the minimum resonant energy of electrons that can be scattered by H-band EMIC waves can extend to tens of keV, although the diffusion coefficients are much lower than those for high-energy electrons. These studies indicate that EMIC waves can effectively influence electrons with energies as low as a few hundred keV. However, these studies cannot distinguish the electron precipitation caused by EMIC waves from precipitation caused by other possible concurrent waves (e.g., whistler-mode chorus and hiss waves). By analyzing the wave number of observed EMIC waves and calculating the minimum resonance energy, Chen et al. [2019] found that during this event, the minimum resonance energy between EMIC waves and electrons is higher than 16 MeV. Thus, the second question we are going to answer in this project is: What energy of electrons are significantly influenced by EMIC waves? A related question is why is the EMIC wave activity in the magnetosphere not always accompanied by the energetic particle precipitation at the conjugate point on LEO orbit?
The precipitation of energetic particles from the magnetosphere can influence the atmosphere. Precipitating energetic electrons are known as a source of nitric oxide (NOx) in the upper mesosphere and lower thermosphere (60 – 140 km). During polar winter, auroral nitric oxide is transported down to the mid-stratosphere (30 – 45 km), with its concentration varying with geomagnetic activity and the dynamical state of the atmosphere. Here, nitric oxides destroy ozone very efficiently. As ozone is one of the key species of radiative heating and cooling in the stratosphere, downward transport of auroral NOx into the stratosphere leads to changes in temperatures and wind fields that can propagate throughout the atmosphere and even affect tropospheric weather systems. Geomagnetic activity is therefore now recommended by the Intergovernmental Panel on Climate Change as part of the solar forcing of the climate system for the Coupled Model Intercomparison Projects-6 experiments for the first time. However, the atmospheric ionization rates needed to drive these model experiments are based empirically on fluxes of precipitating electrons which have a large uncertainty, and recent studies suggest that there may be serious problems with the accuracy of these data. The third question we are going to answer is: Which is energy range of precipitating electrons that have the largest impact on the chemistry and dynamics of the middle atmosphere?
Our project is timely in the following aspects:
- We are going to answer the question raised by an innovative study [Haas et al., 2023].
- Our study will shed light on the puzzling topic about the energy of effective electron precipitation by EMIC waves, which is currently under deep debate.
- New data from operating spacecraft missions (e.g., ELFIN, Lomonosov, Arase) are available.
- New method of including nonlinear effects in global simulations has been introduced by Artemyev et al (2022) but has not been implemented yet.
Project work plan
To address these questions, spacecraft data analysis, numerical simulations and theoretical studies will be carried out synergistically. Our project aims to build upon the research experience of the team members and take full advantage of conjugate observations from space (e.g., MMS, Van Allen Probes, Arase, Cluster, NOAA POES, MetOp, ELFIN, DMSP, Lomonosov) and ground-based observations (e.g., THEMIS ground stations, Chinese Arctic Yellow River Station) in studying precipitation of particles and the related auroral phenomenon. The team members will utilize their expertise in plasma wave theories, satellite data processing, empirical wave modelling, kinetic simulations, and MHD simulations to conduct the research on the scattering mechanisms related to precipitation, including the effects of nonlinear particle scattering. We plan to analyse ground-based and spacecraft observations of plasma, energetic particles, magnetic and electric fields, plasma waves at different frequencies, and aurora images. We will also carry out theoretical analyses using quasilinear and nonlinear paradigms to understand plasma waves and their interactions with charged particles.
To address the first question, we will perform simulations for the same event in Haas et al (2023) and several other events including the effects of ECH waves besides chorus and hiss waves. Currently, there are no models of the wave normal angle of ECH waves due to the difficulty in detecting all 3 electrical components. We will use the observation of ECH waves in those events. We will also include the effects of field line curvature scattering and subauroral polarization streams (SAPs), as well as the effects of nonlinear wave-particle interactions following Artemyev et al (2022).
To address the second question, we will first focus on several typical events, such as the radiation belt dropout event on September 24, 2013, and the EMIC wave events during the conjugate observation periods between Arase and Van Allen Probes (April-May 2017 and September 2017). We will also investigate a longer period lasting several months in 2017, to understand under which circumstances EMIC waves cause rapid loss and precipitation of particles and in which cases the influence of EMIC is not significant. We will investigate ring current and radiation belt dynamics through examination of the challenge events selected by the National Science Foundation Geospace Environment Modelling (NSF-GEM) focus groups. These events have conjugated observations from multiple satellites, which will enable comprehensive investigations of cross-region interactions (Figure 2 shows various satellite trajectories in three storm events). Particularly, we will examine the effects of storm time injection on the ring current and radiation belt dynamics, as well as particle precipitation. We will further perform MHD simulations and kinetic simulations using inner magnetosphere codes to study the dynamics of both fields and plasma, thus providing a global context for the underlying processes. These simulated results will be validated against satellite observations.
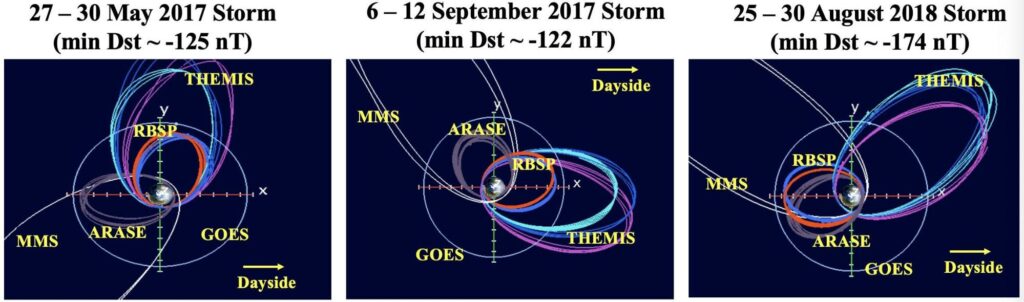
To address the third question, we will perform MHD and kinetic simulations to model electron precipitation driven by wave-particle interactions with chorus, plasmaspheric hiss, hiss in plumes, ECH waves, EMIC waves, and VLF transmitter waves. The precipitated particle fluxes and their energy spectra from the simulations will be validated against data from NOAA POES, ELFIN-L, Lomonosov, and DMSP satellites. Modeled electron fluxes will be used as input in the chemistry-climate model EMAC/EDITh (surface to 220 km, e.g., Sinnhuber et al., 2022) at the top of the model domain. Modeled temperature and nitric oxide will be validated against observations. Case studies will be carried out for a set of Co-rotating Interaction Regions (CIR) and solar Coronal mass ejections (CMEs) driven storms, as well as during quiet geomagnetic periods, to investigate how the different loss processes affect different atmospheric regions.
Schedule of the meetings
We will have monthly teleconferences and two one-week-long meetings at ISSI Bern. These two one-week-long meetings will mainly be in-person but it can be held in a hybrid mode if key team members have difficulties to attend in person. Team members have committed to join the meetings in Bern in-person at the time of proposal submission. Each of these meetings, scheduled to maximize attendance, will involve 11 team members and several students and young scientists. There will also be several attendees joining our in-person meetings using their own funding. During the first three teleconferences, team members will introduce themselves and give presentations about their works relevant to the topic. During these initial telecons, we will define in consultation with team members the structure of the review paper and their possible contributions to its different sections.
The first in-person meeting will be held in October 2024 and the second one in September2025. The exact dates of the meetings will be adjusted depending on specific circumstances and the progress in the resolution of the specific science questions identified in the proposal. We will submit our final report before January 1st, 2026. The goals for the in-person meetings are the following:
2024 meeting
- Discuss the conjugate observations and simulation results for the selected events.
- Discuss the initial results of including ECH waves, field line curvature scattering and SAPs in the simulations.
- Discuss collaborations between team members and outline future research plans.
2025 meeting
- Team members give presentations about their works on all initiated topics.
- They assess the status and final touch-up of the review paper and submit it.
- They discuss future works and possible collaborations.
- Write up papers to summarize the results.
Expected outputs:
- Papers: we will publish a comprehensive review paper about the precipitation of energetic particles from the magnetosphere and the effect of them on the atmosphere. Works about the conjugate observations and simulations will be published in peer-review journals. All these papers will acknowledge the support provided by ISSI.
- Codes: We will make the code to reproduce the paper results publicly available.
- Data: the data used in these papers will also be publicly available.
- Proposals: we will write proposals for future collaboration and potential new satellite mission. The result could also help others in mission planning and specific configuration needed to study the temporal and spatial scales of precipitation.
References:
- Artemyev, A. V., Zou, Y., Zhang, X.‐J., Meng, X., & Angelopoulos, V. (2024). Energetic particle precipitation in sub‐ auroral polarization streams. Geophys. Res. Lett., 51, e2023GL107731.
- Artemyev, A. V., Mourenas, D., Zhang, X.-J., & Vainchtein, D. (2022). On the incorporation of nonlinear resonant wave-particle interactions into radiation belt models. J. Geophys. Res: Space Physics, 127, e2022JA030853. https://doi.org/10.1029/2022JA030853
- Cao, X., Shprits, Y. Y., Ni, B., and Zhelavskaya, I. S. (2017). Scattering of ultrarelativistic electrons in the Van Allen radiation belts accounting for hot plasma effects. Scientific reports, 7 (1), 17719. doi: 10.1038/s41598-017-17739-7
- Capannolo, L., Li, W., Ma, Q., Zhang, X.-J., Redmon, R. J., Rodriguez, J. V., Reeves, G. D. (2018). Understanding the driver of energetic electron precipitation using coordinated multisatellite measurements. Geophys. Res. Lett., 45 (14), 6755-6765. doi:10.1029/2018GL078604.
- Chen, L., Zhu, H., and Zhang, X. (2019). Wavenumber Analysis of EMIC Waves. Geophys. Res. Lett., 46 (11), 5689-5697. doi: 10.1029/2019GL082686
- Haas, B., Shprits, Y. Y., Allison, H. J., Wutzig, M., and Wang, D. (2023). A missing dusk-side loss process in the terrestrial electron ring current. Scientific Reports, 13(1), 970.
- Kennel, C. F., and F. Engelmann (1966), Velocity space diffusion from weak plasma turbulence in a magnetic field, Phys. Fluids, 9, 2377.
- Kasahara, S., et al. (2018), Pulsating aurora from electron scattering by chorus waves, Nature, 554, doi:10.1038/nature25505.
- Li and Hudson (2019), Earth’s Van Allen radiation belts: From discovery to the Van Allen Probes era. J. Geophys. Res: Space Physics, 124, 8319-8315, doi: 10.1029/2018JA025940.
- Miyoshi, Y., K. Sakaguchi, K. Shiokawa, D. Evans, J. Albert, M. Connors, and V. Jordanova (2008), Precipitation of radiation belt electrons by EMIC waves, observed from ground and space, Geophys. Res. Lett., 35, L23101, doi:10.1029/2008GL035727.
- Omura, Y., Y. Katoh, and D. Summers (2008), Theory and simulation of the generation of whistler‐mode chorus, J. Geophys. Res., 113, A04223, doi:10.1029/2007JA012622.
- Orlova, K., Shprits, Y., & Spasojevic, M. (2016). New global loss model of energetic and relativistic electrons based on Van Allen Probes measurements. J. Geophys. Res: Space Physics, 121, 1308-1314. https://doi.org/10.1002/2015JA021878.
- Sinnhuber, M., Nieder., H. and Wieters, N. (2012), Energetic particle precipitation and the chemistry of the mesosphere/ lower thermosphere, Surv. Geophys., 33, 1281-1134, doi: 10.1007/s10712-012-9201-3.
- Sinnhuber, M. and Funke, B. (2019), Energetic electron precipitation into the atmosphere, In: The dynamic loss of Earth’s radiation belts, 279-321, Elsevier, Amsterdam, doi: 10.1016/B978-9-12-813371-2.00009-3.
- Sinnhuber, M., et al. (2022), Heppa III Intercomparison Experiment on Electron Precipitation Impacts, J. Geophys. Res: Space physics, 127 (1), e2021JA029466. doi:10.1029/2021JA029466.
- Shprits, Y. Y., D. A. Subbotin, N. P. Meredith, S. R. Elkington. (2008), Review of modelling of loses and sources of relativistic electrons in the outer radiation belt. II: Local acceleration and loss, J. Atmos. Sol. Terr. Phys., 70, 14, 1694–1713, doi:10.1016/j.jastp.2008.06.014.
- Thorne, R. M. (2010), Radiation belt dynamics: The importance of wave-particle interactions, Geophys. Res. Lett., 37, L22107, doi:10.1029/2010GL044990.